Research Interests
Our group is working on a research program Chemical Approaches to Control the Function of Regulatory RNAs supported by an National Institute of General Medical Sciences Maximizing Investigators' Research Award (MIRA R35 GM130207). The research projects of our program focus on using synthetic organic chemistry to explore and modulate structure and function of biologically significant RNA molecules. Research Assistant positions for new graduate students and postdocs are available on all projects.
- Backbone-Modified RNA: Improving the Specificity of RNA Interference and CRISPR
- Sequence Selective Recognition of Double-Stranded Non-Coding RNA via Triplex Forming PNA also supported by National Science Foundation, Chemistry Division (CHE-2107900).
- Targeting SARS-CoV-2 RNA Pseudoknots Using Triplex-Forming Peptide Nucleic Acids supported by National Institute of Allergy and Infectious Diseases (R21 AI165377).
Backbone-Modified RNA: Improving the Specificity of RNA Interference and CRISPR
Supported by an NIGMS Maximizing Investigators' Research Award MIRA R35 GM130207.
RNA-based technologies to control gene expression, such as, RNA interference (RNAi) and CRISPR have become powerful tools in molecular biology and genomics. The exciting potential that RNAi and CRISPR may also become new therapeutic approaches has reinvigorated interest in chemically modifying RNA to improve its properties for in vivo applications. Chemical modifications can improve enzymatic stability, in vivo delivery, cellular uptake, and sequence specificity; as well as minimize off-target activity of short interfering RNAs (siRNAs) and CRISPR associated RNAs (crRNAs). The long-term goal of our research is to develop chemical modifications for optimization of in vivo potential of siRNAs and crRNAs.
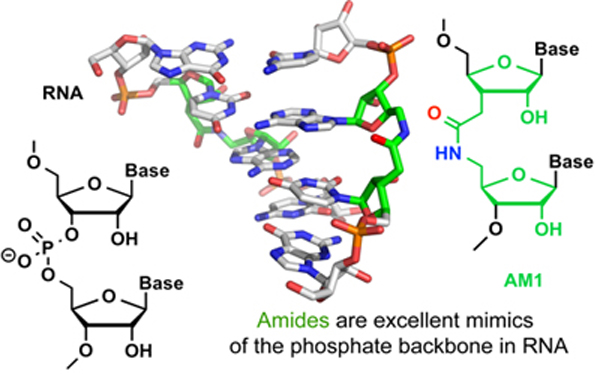
The project builds on our recent discoveries that amide linkage (AM1 in Figure) is an excellent structural replacement for phosphate in RNA. Currently, we are testing further hypotheses that amides may also mimic the phosphate-amino acid interactions in RNA-protein complexes and may significantly improve the properties of siRNAs and crRNAs. The central innovative aspect of this research is in replacing the negatively charged and polar phosphate with the neutral and relatively hydrophobic amide linkage. Our recent results strongly support the hypothesis that replacement of phosphates with amides will be well tolerated in siRNAs and crRNAs. The specific aims of the project are to:
- Study the specificity of siRNAs having individual phosphates of the guide strand systematically replaced with amides. We hypothesize that amide modifications in the guide strand will significantly decrease the microRNA-like off-target activity of siRNAs.
- Study the activity and specificity of crRNAs having individual phosphates of the guide strand systematically replaced with amides. We hypothesize that amide modifications will be well tolerated in the guide strand of crRNA and may increase CRISPR specificity when placed at certain positions.
- Explore novel backbone modifications of siRNAs and crRNAs. Specifically, we are studying how replacement of phosphates with amine, thioamide, formacetal, and related linkages may modulate RNA-protein interactions and optimize the properties of siRNAs and crRNAs.
The overreaching idea is to develop RNA chemical modifications that avoid unproductive electrostatic repulsion and capitalize on productive electrostatic attraction while concurrently enhancing sequence specificity of molecular interactions. This thrust grows out of our recent discoveries that RNA is unusually receptive to chemical modifications that neutralize the negative charge of phosphate backbone. If successful, our research will contribute to addressing key gaps in RNA interference and CRISPR technologies for targeting therapeutically relevant RNAs, and will open doors for development of unique research tools and new therapeutic strategies.
Sequence Selective Recognition of Double-Stranded Non-Coding RNA via Triplex Forming PNA
Supported by an NIGMS Maximizing Investigators' Research Award MIRA R35 GM130207 and NSF CHE-2107900.
The protein-coding mRNA represents only a small fraction of RNA transcribed from genomic DNA. The majority of cellular RNA consists of the so-called non-coding RNAs that play important yet not fully understood roles in regulation of gene expression. Molecular recognition of such regulatory RNAs would be highly useful for fundamental biology and practical applications in biotechnology. However, most non-coding RNAs fold in double-stranded conformations and molecular recognition of such structures is a formidable problem.
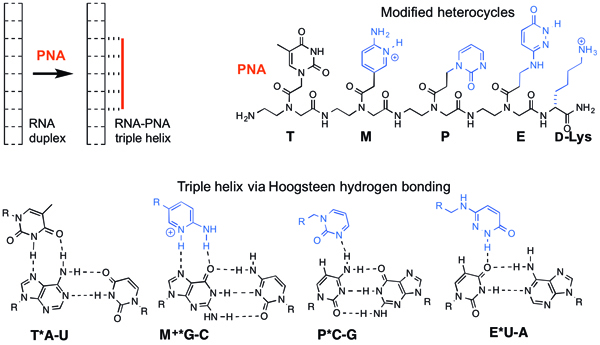
This project builds on our recent discoveries that peptide nucleic acid (PNA) forms highly stable and sequence selective triple helices with double-stranded RNA at physiologically relevant conditions and will test the hypothesis that modified nucleobases (Figure) will allow sequence selective recognition of a vide variety of double-stranded RNAs. The long-term goals of our research are to (1) discover new modes of sequence-selective recognition of double-stranded RNA (dsRNA) and (2) develop novel tools for recognition of and functional interference with biologically relevant non-coding dsRNA. The objectives of this proposal are to:
- Study the triple helical recognition of dsRNA using nucleobase-modified PNA (Figure) at physiologically relevant conditions. We propose that further development and optimization of the modified nucleobases, such as M, P and E, will expand the scope of recognition to sequences common in biologically significant non-coding dsRNA. We will explore the exciting possibility that triple helix formation could be used to recognize any dsRNA sequence.
- Study the conjugation of PNA with cationic peptides and hydrophobic ligands to enhance the cellular uptake of PNA. Poor cellular uptake is the most significant bottleneck limiting the applications and impact of PNA technology. We hypothesize that the cationic peptides and fatty acids will increase cellular permeability of PNA while providing additional enhancement of the triple helix stability by binding selectively in the major groove of RNA.
- Study the use of PNA-RNA triple helices for controlling dynamic RNA structures. We hypothesize that PNA binding will lock the conformation of larger bulges and loops allowing unique studies of biological function of individual conformers. The long-term goal is to develop tools enabling studies on the biological significance of dynamic structures of large RNAs.
Exploring the sequence selective recognition of RNA duplex will advance fundamental knowledge on molecular recognition of nucleic acids. Development of sequence selective RNA binders is important for understanding the biochemistry of non-coding RNAs and may strongly impact fundamental RNA biology and practical applications in biotechnology. Our results suggest that PNA is an unexpectedly well-suited ligand for recognition of biologically important RNA species and has unique RNA over DNA selectivity.
Targeting SARS-CoV-2 RNA Pseudoknots Using Triplex-Forming Peptide Nucleic Acids
Supported by an NIAID R21 AI165377.
SARS-CoV-2, the causative agent of COVID-19, is a positive-sense single-stranded RNA virus in the Coronaviridae family. The RNA of SARS-CoV-2 folds into complex double-helical structures that open the door for novel anti-viral approaches targeting RNA instead of proteins. Positive-sense RNA viruses use the specifically folded RNA structures to highjack the host cell’s machinery, regulate viral replication and mRNA translation, evade antiviral responses, and produce more viral particles. The mechanisms by which SARS-CoV-2 and other coronaviruses control these processes are not well understood. However, highly conserved structural motifs of viral RNA, such as pseudoknots and hairpins, are critical for replication and translation. These motifs act as functional switches of the viral life cycle and are critical for SARS-CoV-2 pathogenesis. In this project, we use triplex-forming peptide nucleic acids (PNAs) to control the conformation and biological function of SARS-CoV-2 pseudoknots. Specifically, we hypothesize that binding of triplex-forming PNAs to SARS-CoV-2 pseudoknots and hairpins will drive the dynamic equilibria of these RNA switches towards one specific structure. For example, an RNA motif in the 3′-UTR of coronaviruses dynamically switches between pseudoknot and double hairpin structures (Figure) that may regulate the switching between replication and translation of SARS-CoV-2. We hypothesize that PNA1 (green in Figure) will drive the switch to the pseudoknot state, while PNA2 (brown in Figure) will drive the switch back to the double hairpin state.
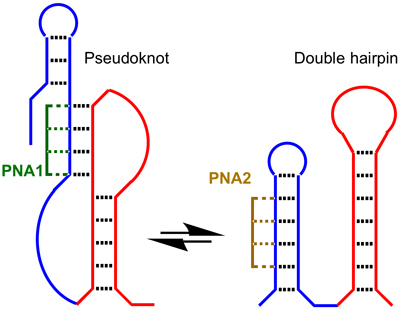
Locking in either of these alternative structures will enable future studies on the biological role of viral RNA switches and evaluate their potential as novel drug targets specific for SARS-CoV-2. The long-term goals are to (1) better understand SARS-CoV-2 biology and pathogenesis and (2) develop new and broad-spectrum therapeutics against multiple coronavirus strains. The specific aims of this project are to:
- Study the conformational control of pseudoknot RNA switches with triplex-forming PNA. We hypothesize that PNAs will bind and stabilize unique RNA conformations thereby controlling the structure and function of RNA switches critical for SARS-CoV-2.
- Study how the PNA binding and RNA switching affects coronavirus biology using a SARS-CoV-2 replicon, containing fluorescent reporters for transcription, translation, and frameshifting. We hypothesize that PNA binding to viral pseudoknots will modulate the frameshifting and alter the ratio of RNA to protein synthesis, which both are critical for efficient SARS-CoV-2 replication and protein expression.
Better understanding of the biological role of viral RNA switches holds tremendous promise to result in innovative antiviral approaches to combat SARS-CoV-2 and other coronaviruses. If successful, the proposed research will develop new tools for studying the biology of complex regulatory viral RNAs, which will have broad and sustained impact on our ability to combat the current and future pandemics.