|
|
Lattice Strain Effects
For
noble metal nanocrystals, lattice strain can generally cause a lattice
contraction featuring a down-shift d-band center, which weakens
the adsorption strength of species on the catalyst surfaces. The
goal of this project is to significantly advance the development of
catalytic sites with lattice strain that exists in several types of
state-of-the-art nanocrystals as model systems. For example, the
lattice-stressed systems can be created by de-alloying binary nano-alloys,
generating property-improved electrocatalysts used for several
applications including but not limited to ORR (one of the fuel cell
reactions), HER and OER (reactions in water splitting), eCO2RR.
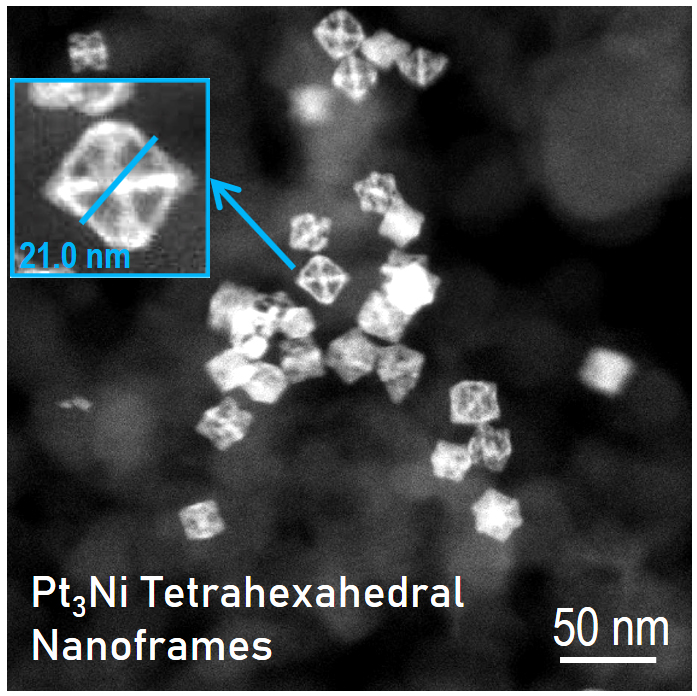
Further reading materials:
Small 17 (46) 2102244 (2021).
10.1002/smll.202102244
Chem. Rev., 121
(2) 736 - 795 (2021).
10.1021/acs.chemrev.0c00436
Facet-Tailored Oxide Catalysts for ORR in Alkaline Media
Recent breakthroughs indicate that some oxides such as spinels
and perovskites could be used as promising electrocatalysts for
ORR (one significant reaction in fuel cells) in alkaline
environments to replace scarce noble metals such as Pt. By
taking advantage of crystal shape-controlled synthesis, this
study centralized on two fronts: facet-dependent effectiveness
and structure-dependent effectiveness. The research activities
include (1) design and synthesis of state-of-the-art oxide
electrocatalysts such as Mn-based spinels with particle shape
control and (2) investigation of the correlation between the ORR
activity/durability and surface feature of the selected
facet-tailored oxide(s).
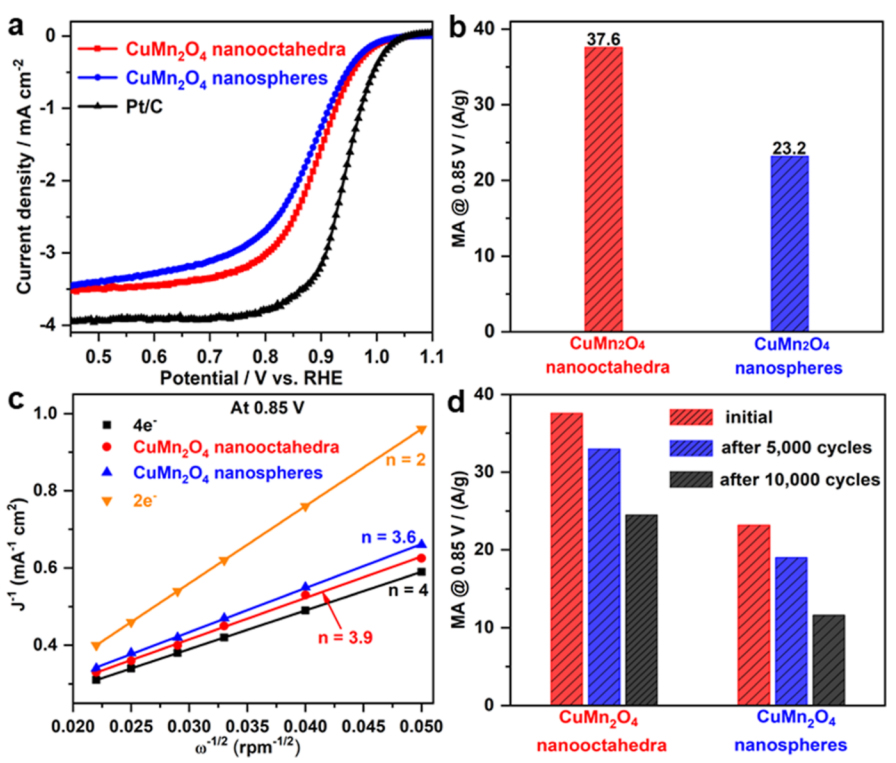
Further reading materials:
ACS Catal., 12 (21) 13663 - 13670 (2022).
10.1021/acscatal.2c03275
|
Single-Atoms Catalysts (SACs) and Single-Atom Alloy (SAA)
Catalysts
Single-atom catalysts (SACs) have become a frontier in catalysis
as an attractive technique with exceptional performance,
offering a promising platform for improving many key
electrocatalytic and catalytic reactions, such as small organic
molecule oxidation and ORR in fuel cells, OER and HER in water
electrolysis, eCO2RR, water-gas shift (WGS) reactions (e.g.,
CO + H2O → CO2 + H2), and
hydrogenation reactions. The supported SACs contain isolated
individual atoms dispersed on, and/or coordinated with, surface
atoms of appropriate supports, which not only maximize the
atomic efficiency of metals but also provide an alternative
strategy to tune the activity and selectivity of
electrocatalytic and catalytic reactions.
In recent years, single-atom alloy (SAA) catalysts have also
been shown to be powerful for a variety of catalytic reactions,
such as selective hydrogenation reactions, dehydrogenation
reactions, oxidation reactions, hydrogenolysis, and coupling
reactions. The creation of SAA catalysts is based on the
deposition of isolated reactive metal adatoms into host metal
surfaces (of a relatively inert metal). The catalytic
performance of SAA catalysts strongly depends on metal-support
interactions and their composition and structure.
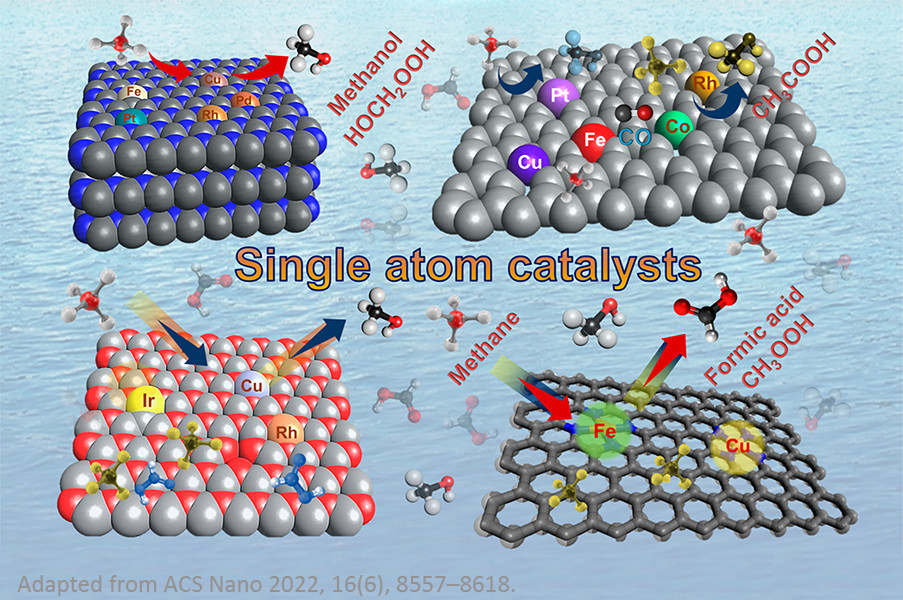
Further reading materials:
Small Struct. 2 2000051 (2021).
10.1002/sstr.202000051
|
Synthesis of 2D Topological Insulators
Topological insulators (TIs), such as Bi2Te3
and Bi2Se3, are
newly discovered quantum
materials. TIs
are bulk insulators for which strong spin-orbit interaction
inverts the orbital character of the conduction and valence
bands at the band edges. Two-dimensional TIs are a remarkable
class of layered materials, exhibiting unique symmetry-protected
helical metallic edge states with an insulating interior. The
realization of various exotic quantum phenomena like the quantum
anomalous Hall effect (QAHE), which is expected to be important
for quantum computing, is rapidly transforming into the
development of realistic materials that can be used to build
novel quantum devices.
Using this insight, we are applying our wet-chemical synthesis
technique to the development of Bi2Te3-based
2D TIs such as tetradymite-type compounds with improved critical
temperature for QAHE, creating a new starting point in the
effort to exploit QAHE via collaboration using a unique natural
heterostructure of intralayer
ferromagnetic and interlayer antiferromagnetic planes
intergrowing with layers of TI materials in the absence of an
external magnetic field.
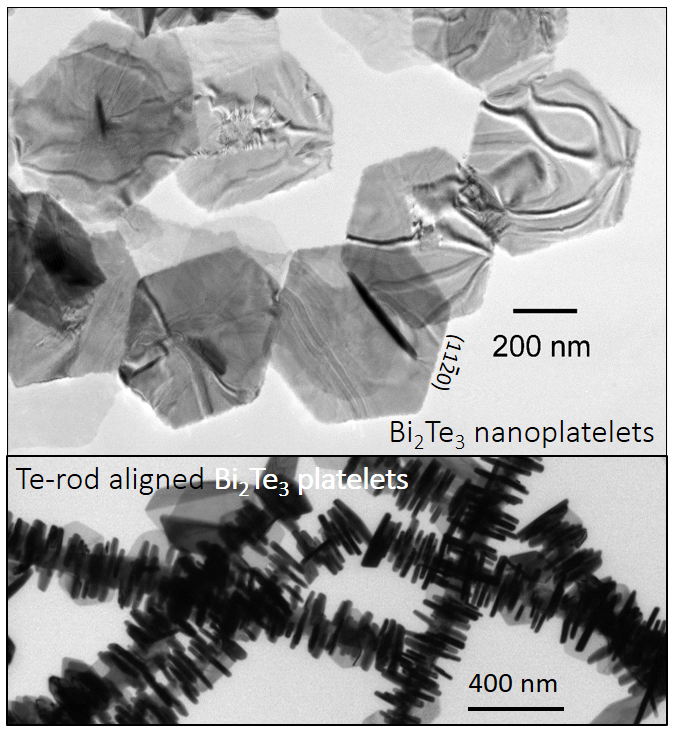
Further reading materials:
J. Phys. Chem. Lett. 8(8)
(2017). 10.1021/acs.jpclett.7b00222
J. Am. Chem. Soc., 127(28), 10112 - 10116 (2005). 10.1021/ja052286j
|
Non-Spherical Assembly
Supercrystals consist of ordered arrays of nanocrystals in 3D or
2D, presenting periodicity in terms of the positions and
chemical compositions of the building blocks. Supercrystals can
be achieved by assembling high-quality nanocrystals that are
synthesized via a wet-chemical approach. A replacement of the
traditional spherical nanocrystals with non-spherical building
blocks could offer some unique characteristics due to the
anisotropic nature when the orientations of the building blocks
are also introduced into the assembly. Meanwhile, the packing
density of the non-spherical nanocrystal-based supercrystals
will be different from that of traditional packing efficiencies
with spherical units. These novel supercrystals could exhibit
not only the building block size- and shape-dependent properties
but also new collective properties that emerge from the optical
and electronic interactions among the non-spherical nanocrystals.
Furthermore, a successful fabrication and understanding of the
superstructures of supercrystals assembled from non-spherical
NCs could result in a rational design to build up novel devices
with specific nano-architecture and meet the demands of various
novel applications. Superstructures of assemblies containing
numerous non-spherical building block systems such as In2O3,
PbS, PbSe, PbTe, Pt3Ni, Pt3Cu2, and
Pt have been studied/reviewed.
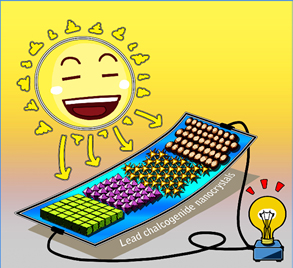
Further reading materials:
NanoToday 5
(5) 390-411,(2010).
10.1016/j.nantod.2010.08.011
Chem. Asian. J. 6
(5) 1126 -1136, (2011). 10.1002/asia.201000937
Acc. Chem. Res. 46 (2) 191-202, (2013). 10.1021/ar200293n
Nano Res., 8
(8), 2445-2466, (2015). 10.1007/s12274-015-0767-1
J. Am.
Chem. Soc. 136(4) 1352-1359, (2014). 10.1021/ja408250q
Nano Lett. 12 (8) 4409-4413, (2012). 10.1021/nl302324b
J. Am. Chem. Soc., 130 (22)
6983-6991, (2008). 10.1021/ja078303h
J. Am. Chem. Soc., 134(34) 14043-14049, (2012). 10.1021/ja304108n
Nano Lett. 11 (7) 2912-2918, (2011). 10.1021/nl201386e
Nano Lett. 17
(01),
362-367, (2017). 10.1021/acs.nanolett.6b04295
|
Pressure-Induced Phase Transition
The property of materials could vary greatly under pressure. For
example, we have uncovered that the semiconductor PbTe has a
high-pressure-tuned metastable structure that can be retained at
ambient conditions. This showed, for the first time, a reversal
of a so-called Hall-Petch relation, relating the structural
stability to particle size (10.1021/nl203409s), raising a
possibility that PbTe semiconductor materials could someday
serve a host of useful technological applications, such as
thermo-electronics, energy conversion, etc. We also focused the
pressure-dependent study on hybrid perovskites, a class of
current state-of-the-art photovoltaic materials. We observed
pressure-induced crystallographic transitions and band-gap
tuning of MAPbI3 (MA = methylammonium) through
collaboration with research groups from NTU and CalTech
(10.1002/adma.201705017; 10.1002/anie.201601788). A later
publication also discussed the case of FAPbI3 (10.1021/jacs.8b09316).
Since the pressure-induced band-gap variation significantly
affects the power conversion efficiency, an application of
modest pressure could be sufficient to initiate phase changes
and bandgap adjustments if the hybrid perovskite compositions
are "pre-adjusted" chemically. Such a combined chemical-pressure
strategy may prove valuable in the design of new perovskites for
photovoltaic applications.
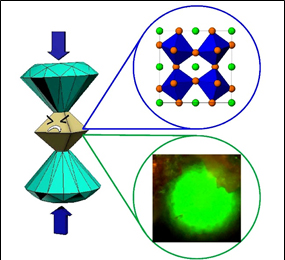
Further reading materials:
Nano Lett.,
2011, 11(12), 5531-5536, 2011. 10.1021/nl203409s
Nanoscale, 8
(9), 5214-5218, (2016). 10.1039/C5NR08291A
Nano. Lett. 13 (8) 3729-3735, (2013).
10.1021/nl4016705
Angew. Chem. Int. Ed. 55
(22) 6540-6544, (2016). 10.1002/anie.201601788
Adv. Mater. 30(2),
1705017, (2017). 10.1002/adma.201705017
J. Am. Chem. Soc. 140(42)
(2018). 10.1021/jacs.8b09316
J. Am. Chem. Soc. 141 (3) 1235 - 1241, (2019).
10.1021/jacs.8b07765
|
|
|